Reactive Materials
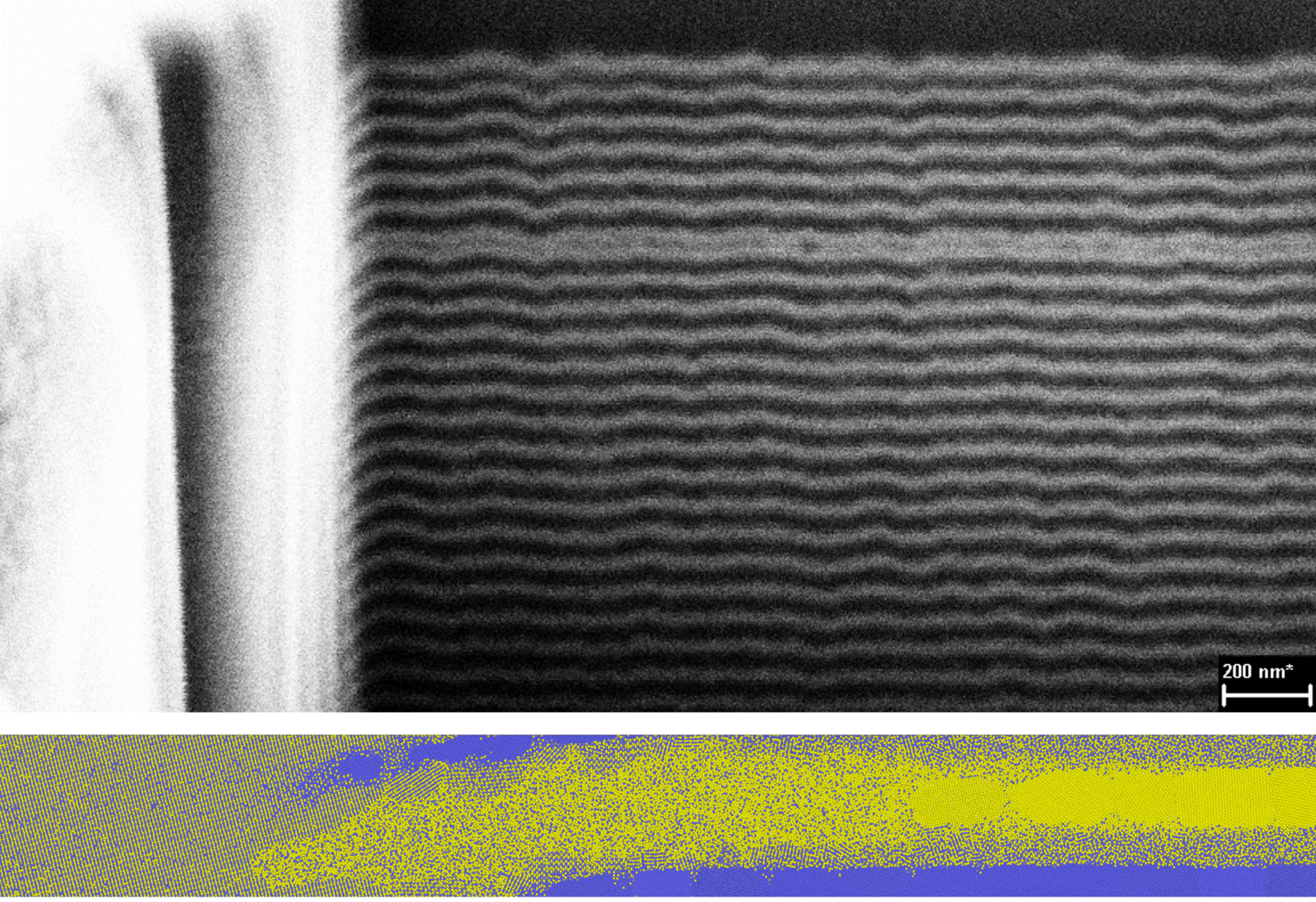
We develop metastable materials with a strong tendency towards intermixing. In particular, we work on reactive multilayers which react in a highly exothermic reaction wave that propagates in a self-sustained fashion in the material. By studying the reactive multilayers both experimentally and computationally, we investigate how the architecture in the nanoscale, composition and other factors influence the propagation of the reaction front. The target of our research is to control, understand and predict the behavior of this type of materials in order to broaden the range of applications in microscale systems and to overcome the limitations of traditional furnace-based healing of metallic materials.
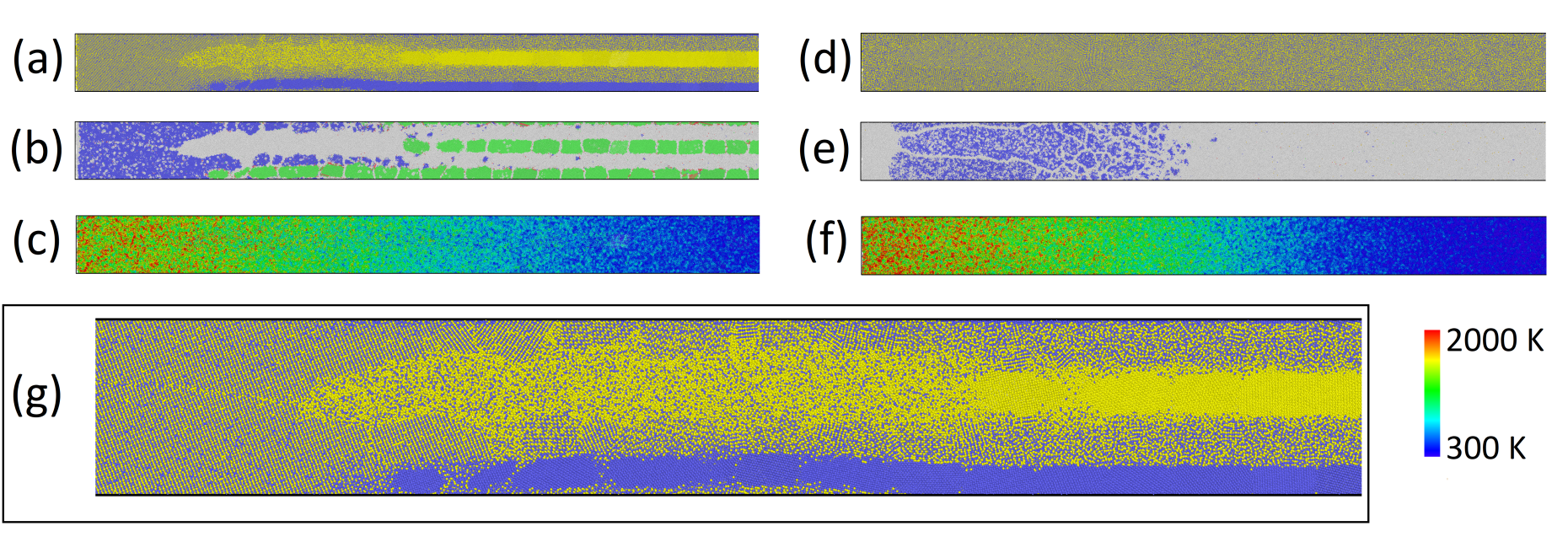
Molecular Dynamics (MD) simulations allow to study reactive multilayers on an atomistic level. While it also has its shortcomings, it allows for great freedom and flexibility with regards to geometry, stoichiometry or crystal structure. On top of the reaction kinetics, which can be studied with MD simulations and compared with experiment, one can also study the underlying diffusion and crystallization mechanisms.
By studying the influence of the crystal structure, the roughness or the role of the premixed interlayer on the front propagation reaction, we bring the systems studied via MD simulations closer to those studied in experiment. This allows us to understand the mechanisms that govern the front propagation. By studying the impact of alloying, varying the stoichiometry or the geometry of the systems, we can develop new ways of controlling the front propagation velocity.
Associated Publications:
- external page Molecular dynamics study of the influence of microstructure on reaction front propagation in Al–Ni multilayers
- external page The influence of premixed interlayers on the reaction propagation in Al–Ni multilayers - An MD approach
- external page An MD-study on changing the elemental distribution and composition by alloying to control front propagation in Al–Ni multilayers
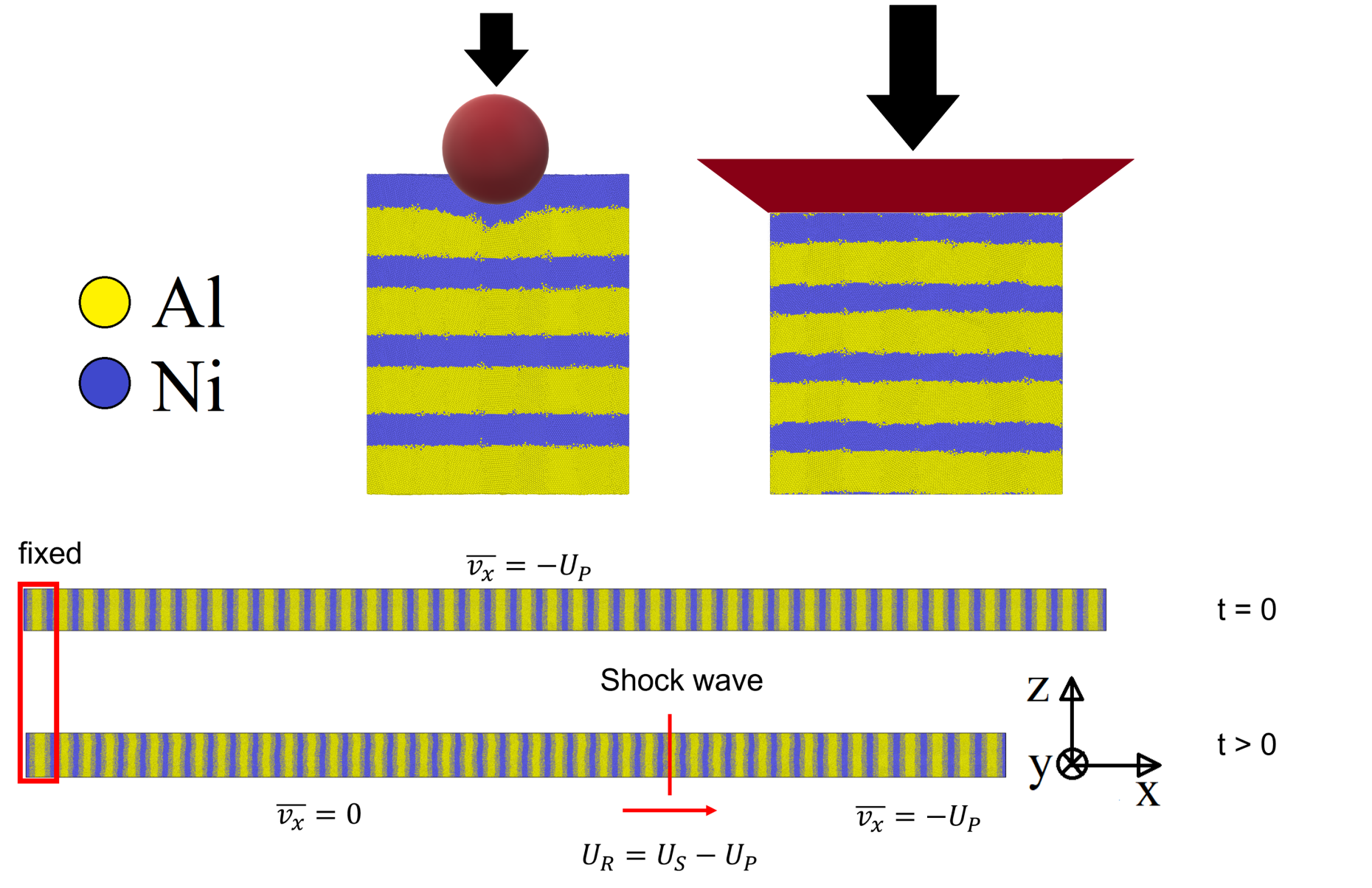
For the integration into various devices, it is important to understand the mechanical properties of the multilayer systems. Using both nanoindentation and uniform bulk compression simulations, we can investigate the dependence of the Young's modulus, the yield strength and the ultimate strength on the bilayer height and the stoichiometry.
Reactive multilayers can also be ignited mechanically. To better understand the underlying mechanisms, we investigate the multilayers via shock compression MD simulations. The simulations reveal not only the dynamic mechanical properties, but also how the reactivity and the phase changes behind the reaction front change with the impact velocity and the bilayer height.
Taking advantage of their nanometer size, on-demand ignition, high temperatures reached, and many other features, these materials find application as intrinsic heat sources in many fields, such as thermal batteries, joining, thin-film healing, and in the microelectronics industry. Each of these applications demands specific propagation velocities and temperature profiles.
Our experimental research is centered on the complex thermal management of Ni/Al reactive multilayers, where we harness architectural design to fine-tune their performance. Magnetron sputtering enables precise control over deposition parameters, consequently influencing the structural design of the multilayers. This approach allows us to experimentally tailor the material's properties to meet the specific requirements of different applications, expanding the range of potential uses for reactive multilayers.
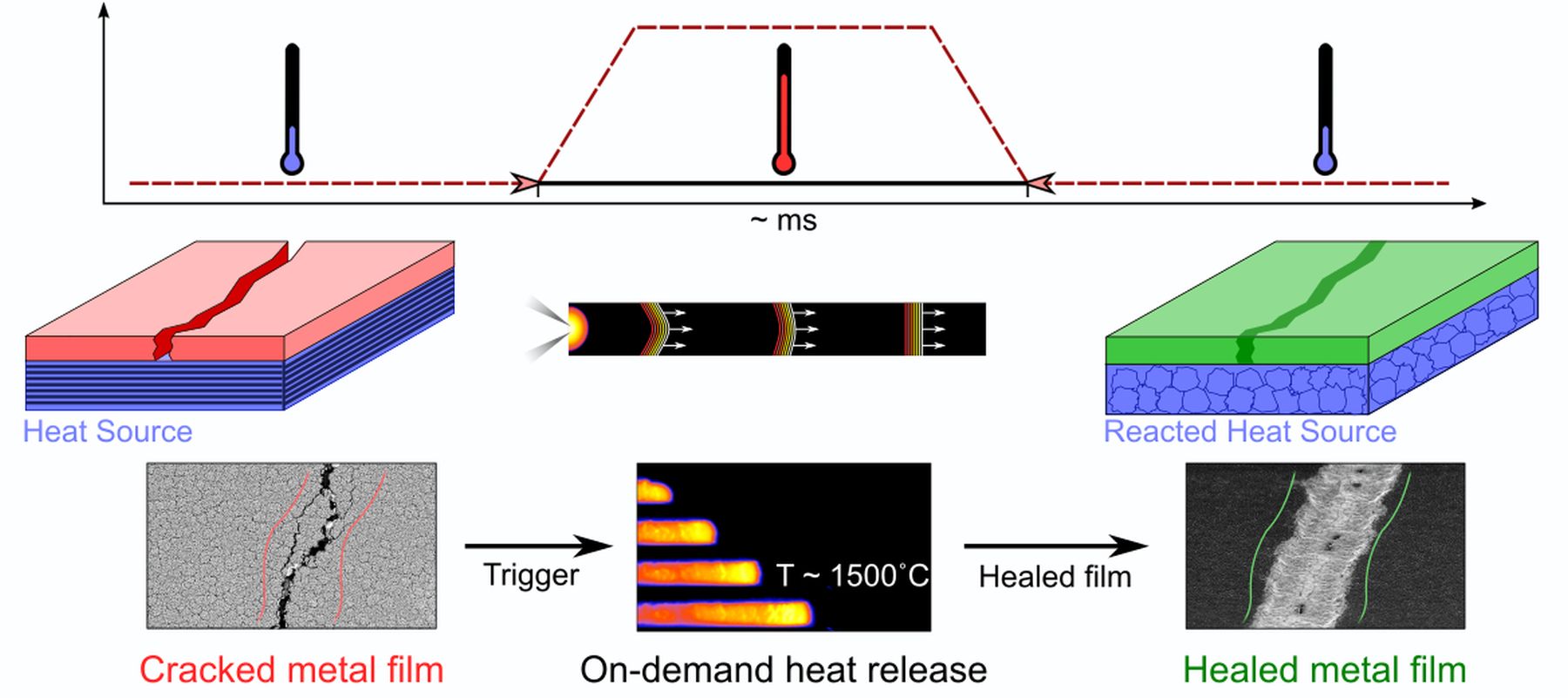
The practical utility of self-healing metals has conventionally been limited by the requirement for external heating to initiate the repair process. In this work, we introduce a new concept for on‐demand healing of metal film that does not rely on external annealing.
Using exothermic Ni/Al multilayers as latent on‐chip heat sources, healing of copper and gold thin films within a few microseconds is demonstrated. The process harvests the heat carried by a self‐sustained solitary heat wave. This methodology holds considerable promise for the realization of authentic self-healing metal thin film systems. Further experimental studies primarily concentrate on the implementation of the system for large-scale deployment.
Associated Publications:
Student Projects
If you are interested in any of these topics for your thesis or semester project, check out our protected page Student Projects. In case you are not enrolled at ETHZ, please contact or .